(→Receptor design) |
(→EGFR transmembrane domain) |
||
Line 57: | Line 57: | ||
<div class="white-box"> | <div class="white-box"> | ||
− | For anchoring in the membrane, a type I membrane protein with defined localization of N- and C-terminus out- and inside of the cell was used. The N-terminal transmembrane α-helix of human EGFR (UniProt P00533, amino acids 622-653) was herefore chosen (see below the topology prediction of EGFR via the [http://www.cbs.dtu.dk/services/TMHMM/ TMHMM 2.0 server for the prediction of transmembrane helices]<ref>Sonnhammer, E. L., Von Heijne, G., & Krogh, A. (1998, July). A hidden Markov model for predicting transmembrane helices in protein sequences. In Ismb (Vol. 6, pp. 175-182).</ref>). A stop-transfer sequence consisting of charged amino acids - being characteristic for type I membrane proteins - was added at the C-terminus, and the sequence was furthermore flanked by a (GGGGC)<sub>2</sub>-linker at the N- and C-terminus, respectively. The addition of a stop-transfer sequence as in the naturally occuring EGFR sequence, as well as the addition of flexible linkers, makes our EGFR-TMD an improvement over the BioBrick [http://partsregistry.org/wiki/index.php?title=Part:BBa_K157002 BBa_K157002], which does not possess either of these elements. | + | For anchoring in the membrane, a type I membrane protein with defined localization of N- and C-terminus out- and inside of the cell was used. The N-terminal transmembrane α-helix of human EGFR (UniProt P00533, amino acids 622-653) was herefore chosen (see below the topology prediction of EGFR via the [http://www.cbs.dtu.dk/services/TMHMM/ TMHMM 2.0 server for the prediction of transmembrane helices]<ref>Sonnhammer, E. L., Von Heijne, G., & Krogh, A. (1998, July). A hidden Markov model for predicting transmembrane helices in protein sequences. In Ismb (Vol. 6, pp. 175-182).</ref>). A stop-transfer sequence consisting of charged amino acids - being characteristic for type I membrane proteins - was added at the C-terminus, and the sequence was furthermore flanked by a (GGGGC)<sub>2</sub>-linker at the N- and C-terminus, respectively. The addition of a stop-transfer sequence as in the naturally occuring EGFR sequence, as well as the addition of flexible linkers, makes our EGFR-TMD an improvement over the BioBrick [http://partsregistry.org/wiki/index.php?title=Part:BBa_K157002 BBa_K157002], which does not possess either of these elements. |
− | [[File:Receptor TMD 002.png|thumb|center|850px|'''Figure 4: A) Multiple sequence alignment''' of human EGFR (see [http://www.uniprot.org/uniprot/P00533 UniProt P00533]), the EGFR transmembrane domain BioBrick from the registry ([http://partsregistry.org/wiki/index.php?title=Part:BBa_K157002 BBa_K157002] and the extended EGFR transmembrane designed by us, containing a charged stop-transfer domain as well as linker elements at the C- and N-termini. '''B) Transmembrane domain prediction by the [http://www.cbs.dtu.dk/services/TMHMM/ TMHMM 2.0 server]''', indicating positioning of N- and C-termini out- and inside of the cell, respectively, as well as predicting functionality of transmembrane regions. '''C)''' Schematic depiction of the C-terminal stop-transfer sequence of the receptor.]] | + | [[File:Receptor TMD 002.png|thumb|center|850px|'''Figure 4: A) Multiple sequence alignment''' of human EGFR (see [http://www.uniprot.org/uniprot/P00533 UniProt P00533]), the EGFR transmembrane domain BioBrick from the registry ([http://partsregistry.org/wiki/index.php?title=Part:BBa_K157002 BBa_K157002] and the extended EGFR transmembrane designed by us, containing a charged stop-transfer domain as well as linker elements at the C- and N-termini. '''B) Transmembrane domain prediction by the [http://www.cbs.dtu.dk/services/TMHMM/ TMHMM 2.0 server]''', indicating positioning of N- and C-termini out- and inside of the cell, respectively, as well as predicting functionality of transmembrane regions. '''C)''' Schematic depiction of the C-terminal stop-transfer sequence of the receptor.]]</div> |
==Other receptor elements== | ==Other receptor elements== |
Revision as of 22:53, 18 October 2016
Receptor design highlights
Think before you ink may be a popular slogan by tattoo adversaries, but it has also been perfectly applicable to creating our synthetic biology approach of bioprinting. In the following, we are going to explore the thought processes that went into the design of the receptors that allow us to embed eukaryotic cells into a stable, three-dimensional and user-definable matrix immediately upon printing.

Herefore, we built two kinds of receptor constructs, both on their own being able - when expressed in mammalian cells - to cross-link cells for our very own approach of bioprinting.
1) A receptor presenting an extracellular biotin acceptor peptide that is endogenously biotinylated by a coexpressed biotin ligase (BirA), thus presenting biotin molecules on the cellular surface, allowing it to react with streptavidin in the printing solution.
2) Receptors presenting an extracellular, monomeric or single-chain avidin variant.
Thus presenting avidin on the cell surface, cells are able to interact with a co-injected biotinylated linker peptide. With this linker being connected to several cell surface avidins as well as streptavidin in the printing solution, a protein matrix is created that embeds cells and allows precise positioning of cells in a three-dimensional manner.
The avidin derivates fused to our receptor, by design, allow the functional fusion of the otherwise essentially tetrameric avidin molecule with our receptor. Two different variants were hereby used: The so-called enhanced monomeric avidin, a single subunit avidin that is able to bind biotin as a monomer, and single-chain avidin, which resembles the naturally occuring avidin tetramer but only consisting of a single ppolypeptide-chain.
Both receptors generally mediate the same purpose: By interacting with streptavidin in the printing reservoir - either directly by presenting biotin (biotin acceptor-peptide) or indirectly via binding to a biotinylated linker (single chain-avidin variants), cells are being cross-linked due to the polyvalent binding of streptavidin molecules in the printing solution to several cellular biotin groups at once.
The incredible journey: signal peptides and protein targeting
Essential to a protein's function is not only its activity or binding properties, but also its presence at the right time, at the right place - in our case, the right place being the cell surface. One of the most ubiquitous elements for protein targeting is the signal peptide, which enables the translocation of proteins to the ER, from where their journey may continue to their place of function. Proteins containing a signal peptide include transmembrane proteins, secreted proteins, proteins of the ER itself, proteins of the Golgi apparatus and several more.[1] For our project, signal peptides constitute a crucial part for many constructs, being used for targeting of transmembrane proteins to the cell surface (receptors), targeting proteins to the ER (BirA) and the secretion of proteins into the surrounding medium (luciferase assays for the quantification of expression levels).

The hydrophobic signal peptide (also called start-transfer sequence) usually consists of less than 30 amino acids [3] and is recognized by the signal recognition particle upon translation at the rough ER, mediating the co-translational translocation of the nascent peptide chain into the ER.[4]
During this project, three different signal peptide constructs are being tested in order to determine the one resulting in the highest expression levels:
- The EGFR signal peptide was taken from the iGEM parts registry ([http://parts.igem.org/Part:BBa_K157001:Design BBa_K157001]) and combined with a BioBrick containing the CMV promoter sequence ([http://parts.igem.org/Part:BBa_K747096 BBa_K747096]) via the RFC10 cloning standard.
- The BM40 and Igκ signal peptides were designed by us and synthesized under the aspect of adding additional spacing and functional elements to the 5'-UTR of the constructs, and combined with a BioBrick containing the CMV promoter sequence ([http://parts.igem.org/Part:BBa_K747096 BBa_K747096]) via the RFC10 cloning standard.
The EGFR signal peptide taken from the parts registry is constructed in a way that the signal peptide ORF immediately follows the RFC10 cloning scar after the CMV promoter, thus resulting in a very short 5’ untranslated region (UTR) of the transcribed mRNA. The combination of the CMV promoter with the synthesized BM40 and Igκ signal peptides allows for a considerably longer 5’-UTR of the resulting mRNA - additionally allowing them to contain a full Kozak consensus sequence by design. The Kozak sequence is recognized by the ribosome as a translational start site; this element missing or deviating from the consensus sequence may considerably decrease translation efficiency.[5] For the EGFR signal peptide construct, a Kozak sequence is not present, as it would have to preceed the start codon ATG - a position which is occupied by the RFC10 cloning scar.
Since both the distance from the promoter to the open reading frame as well as the Kozak consensus sequence are considered crucial parameters for expression levels[6], BM40 and Igκ signal peptide constructs are likely to resut in increased expression levels compared to proteins containing the EGFR signal peptide from the parts registry.
In order to predict whether our designed signal peptides would be functional, we used the [http://www.cbs.dtu.dk/services/SignalP SignalP] server, which allows to make theoretical predictions about signal peptide functionality and cleavage.[7]
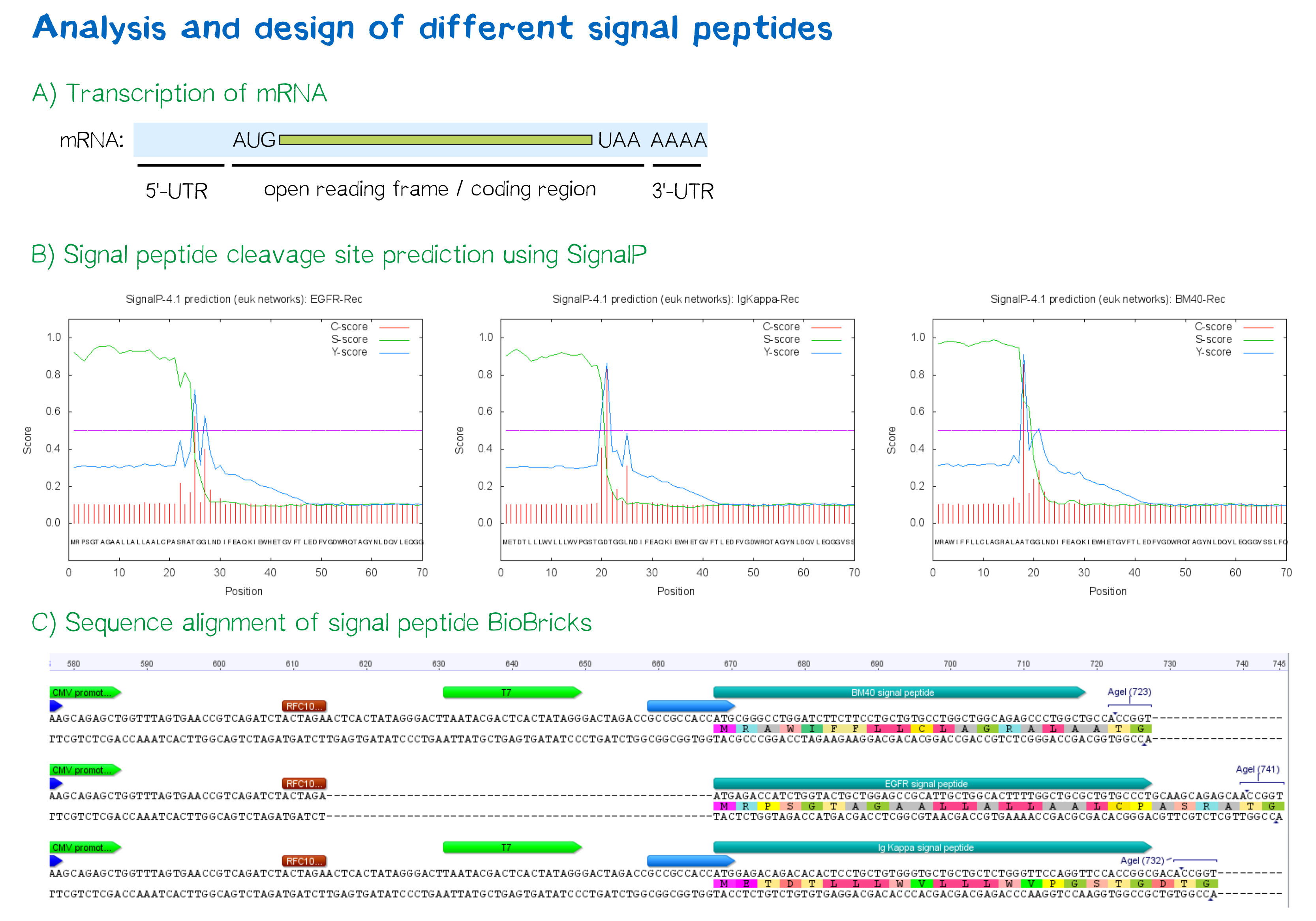
Other receptor elements: stability and detection
Apart from the functional parts that mediate the (strept)avidin-biotin interaction, other elements in the receptor were designed to make sure it is optimally translocated to the membrane, as stable as possible, and easily detectable.
EGFR transmembrane domain
For anchoring in the membrane, a type I membrane protein with defined localization of N- and C-terminus out- and inside of the cell was used. The N-terminal transmembrane α-helix of human EGFR (UniProt P00533, amino acids 622-653) was herefore chosen (see below the topology prediction of EGFR via the [http://www.cbs.dtu.dk/services/TMHMM/ TMHMM 2.0 server for the prediction of transmembrane helices][8]). A stop-transfer sequence consisting of charged amino acids - being characteristic for type I membrane proteins - was added at the C-terminus, and the sequence was furthermore flanked by a (GGGGC)2-linker at the N- and C-terminus, respectively. The addition of a stop-transfer sequence as in the naturally occuring EGFR sequence, as well as the addition of flexible linkers, makes our EGFR-TMD an improvement over the BioBrick [http://partsregistry.org/wiki/index.php?title=Part:BBa_K157002 BBa_K157002], which does not possess either of these elements.

Other receptor elements
Moreover, the receptor contains three functional elements for its detection:
- The intracellularly located red fluorescent protein mRuby 3 for detection of the receptor via fluorescence microscopy,
- an extracellular epitope domain for immunochemical detection via A3C5-antibody fragments and
- an intracellular Strep-tag II for the detection and purification via immunochemical methods.
The vector furthermore contains the CMV promoter for overall high expression levels of the receptor, resulting in a high-avidity-interaction. At the 3'-end, the construct contains the poly-adenylation signal of human growth hormone (hGH) for functional polyadenylation of the transcribed mRNA.
The final construct
The final expression construct for the eukaryotic cell receptor is shown belown for the receptor with the biotin acceptor peptide (BAP). Other constructs differ from this one by not encoding the eIF4G1-IRES as well as not encoding the biotin ligase BirA. Instead of the biotin acceptor peptide, those constructs encode a monomeric or single-chain avidin variant.
References
- ↑ Rapoport, T. A. (2007). Protein translocation across the eukaryotic endoplasmic reticulum and bacterial plasma membranes. Nature, 450(7170), 663-669.
- ↑ Taken from Alberts, B., Bray, D., Hopkin, K., Johnson, A., Lewis, J., Raff, M., ... & Walter, P. (2010). Essential cell biology. Garland Science.
- ↑ Walter, P., Ibrahimi, I., & Blobel, G. U. N. T. E. R. (1981). Translocation of proteins across the endoplasmic reticulum. I. Signal recognition protein (SRP) binds to in-vitro-assembled polysomes synthesizing secretory protein. The Journal of Cell Biology, 91(2), 545-550.
- ↑ Keenan, Robert J., et al. "The signal recognition particle." Annual review of biochemistry 70.1 (2001): 755-775.
- ↑ Kozak, M. (1989). The scanning model for translation: an update. The Journal of cell biology, 108(2), 229-241.
- ↑ Kozak, M. (1987). An analysis of 5'-noncoding sequences from 699 vertebrate messenger RNAs. Nucleic acids research, 15(20), 8125-8148.
- ↑ Petersen, T. N., Brunak, S., von Heijne, G., & Nielsen, H. (2011). SignalP 4.0: discriminating signal peptides from transmembrane regions. Nature methods, 8(10), 785-786.
- ↑ Sonnhammer, E. L., Von Heijne, G., & Krogh, A. (1998, July). A hidden Markov model for predicting transmembrane helices in protein sequences. In Ismb (Vol. 6, pp. 175-182).